
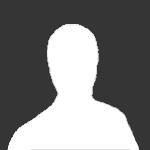
Chopsticks
Members-
Posts
9 -
Joined
-
Last visited
Recent Profile Visitors
The recent visitors block is disabled and is not being shown to other users.
Chopsticks's Achievements

Lepton (1/13)
2
Reputation
-
Does color filter change the frequency of light?
Chopsticks replied to vibhorsethi's topic in Quantum Theory
Most nitpicky: a photon is partially defined by its' frequency. To change the frequency the photon must interact with something, which destroys the old photon and creates a new photon of different frequency. As used more in practice, if you want to change the frequency you can use nonlinear optics A frequency doubling crystal is probably the simplest case. You shine a infrared or red laser into the crystal, you get out a laser at twice the frequency, most often green (and other garbage light you don't want). For LED bulbs, the first type had separate red, green, and blue LEDs inside, so their spectrum would have 3 spikes not a smooth curve. So you could use a very narrow filter to knock out all of the red, green, or blue. Possibly it could be narrow enough that the filter would look transparent in sunlight. -
material that has fast light-sensitive transparency/opacity?
Chopsticks replied to wosoka's topic in Classical Physics
So, you need very fast transition, reversibility, and locality (? whatever word describes a bulk material where only one part changes). I suspect you won't find anything that can do completely clear to completely opaque; if you can't get 100%/0% what kind of percentages can you tolerate? My first google was reversible light sensitive polymers, but couldn't find any speed information. Closest I saw was 100s each way. I guess those are the photochromic films you mentioned in the OP I do know something that will do fast and reversible, and somewhat local (the version I know creates a interference pattern, not a single spot). Look up "optical kerr effect" and maybe "four-wave mixing" on wikipedia. The version I worked with, you have a liquid crystal "capacitor" - crystal is in something transparent, with electrodes to apply a voltage difference to the front and back sides. Bias that with a few hundred volts - almost no current unless something blows up. Then you can do things like beamsplit a laser and recombine at the cell to get a dark interference pattern. For what you want, I think you can just shine a single powerful laser at the cell, and the optical kerr effect will happen at that point. Now, this bends the light instead of absorbing it so you need a beam dump to one side, but that should still give you a dark spot on an image. (in some respects this is similar to a biased diode. the voltage is almost enough to switch the liquid crystal, and the light hitting a spot adds energy to push that area to switch) Reviewing, maybe not quite what you wanted. The cell wouldn't necessarily darken, but if you projected an image through the cell the same time as the laser, then the image would come out with a dark spot at the laser location. Obligatory safety reminder: Lasers powerful enough to do something interesting can blind you or do other nasty things, even IR lasers, and any scattered light is still rather dangerous. Wear your laser safety goggles. -
generating electricity with fusion explosives
Chopsticks replied to trevorjohnson32's topic in Classical Physics
While the OP's designs are wouldn't work because of cleanup costs and speed of repetition (but would work in Dwarf Fortress), #1 is pretty similar to Inertial Confinement Fusion Wikipedia. Squish a small pellet until it fuses, extract the heat into water, use that to drive a turbine, repeat the cycle many times a second. Steps 2 & 4 are the problems right now. You need to confine the plasma without damaging the container, and extract the energy somehow without letting the plasma escape. And you have to repeatedly and automatically get a new pellet into the exact right spot within a um or so, in only a few ms. -
why do two objects fall same rate in a vacuum
Chopsticks replied to trevorjohnson32's topic in Classical Physics
Folks upthread covered the theory and thought experiments, now here's the line of reasoning leading to a practical experiment of the theory (used in at least one science museum, and IIRC was Galileo's real experiment) Objects fall at the same speed. Okay, so they should still fall at the same speed if they fall next to a vertical surface, since they aren't touching it. Let's make the vertical surface really smooth (a good sheet of ice say), then tilt it just a little from vertical, and drop the objects from the top. The objects still fall down, but the surface deflects them sideways. Since it is a frictionless surface it won't change the speed, just direction 2a Changing the direction means you change the velocity from totally vertical to a mix of horizontal and vertical. This means things fall more slowly, so you can see the experiment without a high speed camera. Make the angle flatter and flatter, you get the experiment of two weights sliding down a low hill In the real world, you want to make your objects as similar as possible so they are equally affected by friction, and by air resistance. Experimental setup. A nice smooth plank, one end on the ground and the other liftable to various heights. Two same-size empty boxes that are smooth on the bottom (some of those smooth plastic furniture sliders on the bottom maybe). Put one pound of anything in one box, two pounds of anything in the other. Slide them both down the slope. Alternatives could be spheres or cylinders - important thing is same size and outer material (bowling ball and an identical bowling bowl hollowed out on the inside, car tire with and without some weight evenly distributed around the inside so maybe a tire with and without a hub) Adam Savage & Vsauce built a really nice example of this kind of experiment setup. Theirs demonstrated different types of hill, but could be used for this experiment if you added weight inside one of the rolling things, maybe hollowed out #2 and kept #3 unchanged. Then start all 3 objects at the same height. Youtube link: Adam savage's slide -
What color light do we see on the emission spectrum from an element?
Chopsticks replied to alpha6's topic in Chemistry
Been there, did the experiment, got the eyestrain. The OP seems to be smushing together two different "ways of looking" at the light. First off, you have a burning sample (or a gas sample in capsule with high voltage). That glows and you see a single color. Purple is the one I most remember, hydrogen or one of the noble gases. That color is all the wavelengths the sample is emitting, mixed together. So that's "look one". Now, let's put a prism between you and the glow. You now see a set of bright lines, each line being a single wavelength., and so a single color (it is possible that you will still see a line the same color in part one, but I think that is only if the purple spectral line is much much brighter than any others) Oh, and a word of warning with that page of spectra. If you do the experiment, you do not see "dim background full spectrum with bright lines". You see only the lines. So those images are a little misleading. The main page doesn't mention it, but if you click on a spectra it includes the disclaimer... -
Websit on this Cornell should be pretty reliable. I would have thought brownish if it had a lot of iron. But basically rock-colored. Looks like the first link in each section is real-color, others are grayscale or false colored.
-
Wavelength, frequency, and phase velocity, and how a signal spreads over distance, if you pick one the others are basically fixed. C=f/w, velocity depends on frequency and refractive index (1.0 almost always), and interaction with antennas/lenses/walls is basically wavelength. What you can vary is modulation and bandwidth. IIRC, bandwidth is independent of carrier frequency, so a 20 kHz audio signal requires 20 kHz whether the carrier is 100 kHZ or 20 Ghz. The practical answer to the initial question is modulation can put the same data in low or high frequency carrier. But, the hardware needed gets much more difficult as the ratio of carrier/mod frequency gets larger. 20 khz signal on 20 Ghz carrier is .00??1% bandwidth, 20 khz signal on 100 khz carrier is 25ish % and much harder. Course, this is still neglecting things like a long wavelength needs long antennae to pick up, some frequencies get absorbed by atmosphere more quickly, and the dynamic range of the signal voltage
-
Propagation of light across a seemingly impassable barrier ?
Chopsticks replied to Dionysus's topic in Classical Physics
Loading weasel words and spherical cows........ done For a perfect fringe pattern, the em field/number of photons in the dark area is 0. (Handwaving: no light there cause it is dark. Mathy: probability function goes to 0 - I think that pattern is sin^2 IIRC) Since there is no light there you can put anything in that region without affecting the light. Adding some reality back in. Only the center of the dark has 0 photons, so you have to use a perfect thread instead of sheet. But, even that is not perfect dark because air diffraction etc. Also, you're right that the sheet must be thinner than the fringe because of edge diffraction and fringe effects. But even given all the caveats, putting anything in the dark region will have a small impact because it's dark there. Now for a more interesting result, put that sheet in the bright areas. Blocking the central bright gives you the same output waveforms, but they will be much dimmer. Blocking other orders of bright fringes will do something, especially if you only block right or left side, but I don't remember what exactly. BTW, cut 'what' beams? You already established that at that plane you have a fringe pattern, aka 'many' beams. -
What we know: Make a bunch of identical particles, send them through your test setup, and measure their position on the far end. The shape of that distribution will depend on the probability function. Now, get rid of all particles except at one position (pinhole is a typical method). Put a position detector past the pinhole. You'll get a particle distribution of a spherical probability wave emanating from the pinhole. Before the pinhole you have one probability distribution. After the pinhole there is a different distribution. So, there was a waveform collapse at the pinhole. (I suspect I'm mashing two different experiments together, but should still be true). Whether that collapse is physical depends how you define physical. My hypothesis is that, whatever "collapse " ends up being, it will be the point where QM and relativity end up meeting. QM interaction where one thing has "enough" mass or something.