
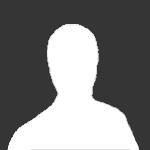
bangstrom
Senior Members-
Posts
309 -
Joined
-
Last visited
Content Type
Profiles
Forums
Events
Everything posted by bangstrom
-
I am not ignoring previous comments and I acknowledge that energy occurs as quanta and it is absorbed in discrete points but I find this feeble evidence to say that light is ever a particle. If photons exist as particles, there must be some evidence for their presence besides the two examples mentioned. Light is quantized because it is emitted and absorbed in quanta determined by the energy levels of the electrons within atoms. Light arrives at a single atom because light is a transverse wave and transverse waves don’t split, spread, or lose energy as you suggest. Separate transverse waves or scalar waves may diverge with distance but individual transverse waves remain intact. Localized energy is not a property unique to particles. I also find it convincing that a two-way wavelike connection is established between an atom at the source and an atom at the sink before a quantum of light energy can be transferred from one to the other so there is no such thing as a miss or a scatter. If powerful laser beams cross, there is no photon scatter from collisions as would be expected if photons were particles. Even simple things like the Dirac three polarizer experiment are best explained by considering light to be a wave rather than a particle and there are recent quantum experiments that can’t be explained with light as a particle. The Afshar experiment was an attempt to identify photons as particles and it failed to do so. The experiment was thoroughly dammed in peer review as error prone but Flores repeated the experiment with all the sources of error corrected and got exactly the same result- waves but no particles. Light appears to act if it is prescient of its destination and Hugo Tetrode explained in 1922 how charged particles must establish a two-wave wavelike connection before they can exchange a quantum of light energy. This is not consistent with light as a random emission that wanders until it hits a target. Wheeler and Feynman also came to the same conclusion about a two way connection with their overly complicated Absorber theory and the W-F theory evolved into John Cramer and Ruth Kastner’s present day Transactional Interpretation of Quantum Mechanics and N.”Viv” Pope’s and Anthony Osborne’s Angular Momentum Synthesis. The latter pair were adamant about dropping the word “photon” from their writings so as not to confuse anyone with old images of the photon as a space traveling particle. I see no reason or occasion to consider light as a particle and it appears to be an obstruction to understanding how light works. This two-way connection is central to contemporary theories of light such as the John Cramer and Ruth Kastner’s “Transactional Interpretation of Quantum Mechanics” or the Pope-Osborne Angular Momentum Synthesis. As for some old business. I was told more than once that a one-slit diffraction pattern is not an interference pattern. This was stated as a matter of fact with no explanation except that it was impossible. I explained how it is possible and considered the matter concluded until I mentioned again that a one-slit diffraction pattern is an interference pattern. That was too much “not listening” and the thread was closed. The problem is that anyone with a home-made slit and a laser pointer can get a perfectly good interference pattern from a single slit and I have done so many times as have many others so how can it be impossible? I know where the idea comes from because the nearly unanimous consensus among physicists is that it is impossible because the broad band observed when light passes through a single slit does not show any of the fine bands of light and dark characteristic of an interference pattern. What gives? For one thing, if your single slit is wide, like more than a wavelength light, and your detector is the side of the kitchen refrigerator, it is easy to see that the diffraction pattern lies of the same plane as where you would expect an interference pattern to fall. If you look beyond the end of the line in the center of the pattern you can see smaller bands of interference. There may only be two side bands visible, but if you make the slit narrower, the long band in the center becomes shorter and less apparent while the bands to the side move inward and become brighter. As the slit narrows, the diffraction looks more and more like an interference pattern. It becomes apparent that the bright band in the center is just one band of a typical looking interference pattern- which it is. The physicists must be looking at just the long bright band in the center thinking it’s the whole diffraction pattern when it is only a single band of an interference and one would not expect to find interference bands within a single band. Here is a quote from Sabina Hossenfelder and she explains this in detail. The bolded letters are mine. http://backreaction.blogspot.com/search?q=quantum+eraser+experiment “The interesting thing about the double-slit experiment is that if you measure which slit the particles go through, the interference pattern disappears. Instead the particles behave like particles again and you get two blobs, one from each of the slits. Well, actually you don’t. Though you’ve almost certainly seen that elsewhere. Just because you know which slit the wave-function goes through doesn’t mean it stops being a wave-function. It’s just no longer a wave-function going through two slits. It’s now a wave-function going through only one slit, so you get a one-slit diffraction pattern. What’s that? That’s also an interference pattern but a fuzzier one and indeed looks mostly like a blob. But a very blurry blob. And if you add the blobs from the two individual slits, they’ll overlap and still pretty much look like one blob. Not, as you see in many videos two cleanly separated ones.” Sabrina Hossenfelder Oct, 2021 If Hossenfelder calls the one-slit diffraction pattern an interference pattern, that’s good enough for me.
-
You asked if there are any instantaneous natural processes and I mentioned entanglement. As for your drawing with a stream, I didn’t see anything instantaneous. You can watch a leaf in a stream flow up and over a hydraulic jump and that’s not instant. There is also a static energy to pressure gradient but a gradient isn’t instant either. What else is there to say? I don't see how anyone could possibly observe the time it takes two particles to become entangled. The "instant" I had in mind involves performing a measurement on one entangled particle and then timing how long it takes for the other paired particle to be influenced. The Chinese were perhaps the most recent to measure this with photons and they measured the time to be something like four orders faster than light. That could qualify as instant or too fast to measure.
-
Can you give us some examples where interactions between light and matter can't be explained as a wave. The photoelectric effect has been discussed and the discrete nature of photon termination can also be explained as a wave without recourse to the simplistic analogy of particle impact. I don't find either of these as convincing evidence for the particle nature of light or am I missing something?
-
It is my understanding that an atom gains energy when an electron jumps from a lower energy orbit to a higher orbit and one should not think of an electron in orbit about the nucleus as having a position in space as if it were a tiny planet in orbit about a star. Electrons are best thought of as probability waves rather than having a location. I have never looked into the thinking behind the theory, but the speculation is that an electron “quantum leaps” from one orbital to the next without passing through the space between. It simply vanishes from one orbit and appears in another without a transition through either space or time so no time interval can be assigned to how long it takes an atom to gain energy.
-
Light is in the frame of light and that is where the transition takes place. Our perception from afar is not a determining factor. Light may land within the probability predicted by energy, distance, and time. That is based on previous observations of similar events in the past but the same interpretation may not apply to the reference frame of light which lacks a time interval even though the end results are the same. And interpreting light as a particle in this case, isn’t that applying a classical model to a quantum event? And what is a “particle” in the quantum view? “When an energy exchange occurs between, say, two molecules one wonders what is traveling between them. If we don’t know, we say it is a “photon” Giving it a name doesn’t add any knowledge, but it allows us to feel better and we can pretend we know what travels.” Milo Wolff “What we observe as material bodies and forces are nothing but shapes and variations in the structure of space. Particles are just Schaumkommen.” (appearances)- Erwin Schroedinger 1937
-
I still suspect the experiment has a explanation in the polarity. The light reaching the moving detector Ds is both linear and circular because quarter wave polarizers QWP’s have a linear polarizer followed by a circular polarizer so the light reaching the moving detector is both linear and circular and beyond just circular as mentioned in the description. The description doesn’t explain the thinking behind the use of the polarizers or their orientations. That is likely to be found elsewhere but the information in the article is inadequate. Diffraction is interference with bands and all.
-
Q:Surely both photons must be in the same frame to entangle them. A: Not necessarily. But for experimental purposes, they must originate from the same spot if we are to recognize them as entangled. In other words, if we have a single particle, we have no way of knowing if it is entangled elsewhere or not. Q: And if they are in the same frame they must be in the same frame and therefore subject to the same transformations, relative to any other frame. A: Entangled particles have no “location.” We can’t say particle A is here and B is there. The locations are said to be "indefinite" until observed. In other words, a single unobserved particle can entangle spontaneously with another particle anywhere on the same light cone. And they are subject to the conditions of their local reference frame. Q: I simply asked because you originally stated quite clearly. 22 hours ago, bangstrom said: The entire body of quantum identities can be entangled Are you suggesting that say position and momentum are not quantum properties or quantum identities (whatever they may be). ? A: Position and momentum are much the same as location. You can’t assign position or momentum to entangled particles until you can identify which particle is where and their location is indefinite until you observe the particle.
-
That’s right but with linear polarized light the interference is so rapid and random that it is no longer apparent. Also, two linear polarizers at orthogonal angles block light but circular polarizers do not. When you have waves from one edge of a single slit and waves from the other edge and they overlap, the two waves interfere. In the case of a single quantum of light energy, the waves are said to be “probability” waves and the interference builds over time- one spot at a time. The part about linearly polarized light interfering so rapidly and randomly that interference is no longer apparent comes from Fresnel and Arago’s original paper where they introduce their three laws of polarity. The loss of interference does not happen with circularly polarized light which counters Skully and Druhl’s theory that the availability of which-path information destroys interference. In which case, which-path information is a bogus explanation. That leaves polarity as the most likely explanation for the loss of interference.
-
Momentum is observer and reference frame dependent and not part of a particle's identity and only the identity can be entangled. Identity includes such things as charge, spin, or polarity. The experiment you cited is new to me but I like the choice. It is much simpler that what I had in mind and it confirms my view that circularly polarized light makes which-path information available while continuing to produce an interference pattern. The explanation about rapid interference refers to Fresnel and Arago' first law of polarization and their explanation of how linearly polarized beams interact. It does not include circularly polarized light which is quite different. Beams of circularly polarized light do not interfere destructively as do linearly polarized light. When light waves passes by an obstruction, they fan out. They diffract. A single slit has two edges and the diffraction patterns from each edge interfere to produce a diffraction pattern which is characteristic of light as a wave. As described by Skully and Druhl, marking the two light beams with polarization makes which-path information available and destroys interference. Marking the light paths with circular polarization does the same without destroying interference contrary to Skully and Druhl but not Fresnel and Arago.. The linear polarization on the p path of your experiment is lost in passage through the two quarter wave polarizers and circular polarization is imparted. Since the beams are circularly polarized even though orthogonal, they can produce interference.
-
I am sure the polarizers could be placed in front of the double slits but it would require a bit of micro-manipulation to position the polarizers just right because the slits are so tiny. That must be why it is never done that way. The diffraction pattern spreads widely behind the slits so it is much easier to position the polarizers to the rear. Plus you can see what you are doing. It should make no difference if the polarizers are in front of the slits or behind. I think that is what you are saying. The polarization should remain the same and the which-path information should remain the same. The question is, Why should we say the which path information information destroys the diffraction pattern when Fresnel and Arago have a perfectly good explanation for why this should happen. On the other hand, the which-path explanation sounds more than a little like new-age crystal woo so I have my doubts. The part that needs explaining is how which-path information can destroy interference. I have personally tried the experiment with circular polarizers (behind the slits) and found that circular polarizers do not destroy interference but they do provide which-path information just as linear polarizers do. This suggests that the culprit is linear polarization rather than which-path information. I haven’t found any information in the literature about what happens if one substitutes circular polarizers for linear polarizers but I have found some anecdotal reports that circular polarizers destroy interference so the matter is in doubt but I haven’t found any indication in Fresnel and Arago to indicate that it shouldn’t work contrary to the which-path theory. I don’t doubt there are several here that know more about quantum physics than I but my comment is in reference to “a single specific experimental setup.” I am just surprised that the physical setup is not better known.
-
I have no idea how much anyone here understands about delayed-choice quantum eraser experiments. Specifically, Kim et al’s experiment which has become the main reference point for discussions about the topic. From the responses I have read so far, the familiarity about the topic here is little to nothing. I could be totally wrong about that but the experiment has been well discussed and explained better than I could ever do by persons elsewhere and with ample illustrations. It would be an enormous effort for me to reinvent the wheel and explain Kim’s experiment when so many others have already gone to the trouble. That is why I find it necessary to refer to other websites for explanations. Unfortunately, nearly all the explanations elsewhere about the quantum eraser end with, ‘We don’t know how it works.’ I assumed the Kim et al experiment would be common knowledge to someone on a quantum thread and need little explanation. I find that Kim’s experiment can be best understood as a “mostly” classical experiment that can be broken down into individual experiments involving known properties of polarized light. That is my intention and I find it more revealing than the usual attempts to understand the experiments. If anyone is familiar with the usual explanations, they resemble trying to follow the paths of four balls “photons” through the bumpers and flickers of a pin ball machine. In the quantum-eraser experiment, after passing through two slits, the BBO crystal, two more birefringent crystals, two color filters, and a prism, the light emerges into the main body of the experiment as two different beams of light that are both linear and circularly polarized. This is much the same mechanism as with a modern style 3D movie projector that uses two beams of light and combinations of both linear and circular polarizations. Some interpreters of the experiment recognize that the light is linearly polarized but they don’t consider that it is circularly polarized as well and that may be a key to a better understanding. You asked me what was before the slits and I said there was “nothing.” You didn’t ask what was behind the slits. But if you recall, I did mention some of what was behind the slits long ago. There is a BBO crystal and two more birefringent crystals and there is a lot more behind that. The BBO crystal is where the action takes place. Remember ? the part about spontaneous parametric down conversion SPDC. If anyone is curious about quantum physics they should have some familiarity with Kim’s experiment. Judging by the titles of articles of all kinds of woo, even people outside of physics may not know the name of Kim’s experiment but they are aware that such an experiment has been done and they are quite familiar with its implications. The entire body of quantum identities can be entangled but we can only choose to discover one and entanglement is lost. A single observation destroys entanglement. You must have read that into my description because I never said anything about the double slit destroying polarization. Polarization takes place after the light passes through the double slit when the light passes either through a polarizing filter or, in Kim’s experiment, when the light passes through the BBO birefringent crystal. The BBO crystal is behind the double slit.
-
I mean “behind” in the usual sense of the word. The light passes through the double slit and then directly into the BBO crystal. The diagram is complicated, as its explanation, but both can be found in thousands of places on the Internet. Wikipedia is a good place to start. Look for “delayed-choice quantum eraser” and follow the article about half way down to where it says “The experiment of Kim et al. (1999)”. That is where you can find the diagram. Every interpretation I can find is about which-path information but which-path information has nothing to do with the results. It is all about polarization. All the hounds are on the wrong trail.
-
I have the impression that there is a lack of understanding about what the delayed-choice quantum-eraser is or specifically which one I have in mind so I will explain that part in a later post. First, the simplest explanation is that the light passes through both slits as a wave and directly behind the slits there is a BBO crystal. A BBO crystal is a birefringent crystal with optical properties nearly identical to calcite. The light can take one of two possible paths. It can take a direct path known as the “p” path or the slightly angled path known as the “s” path. Either path it takes, the light emerges from the crystal as two parallel beams that are oppositely polarized both linearly and circularly. When light passes through a birefringent crystal one wavelet at a time, it can either take the p path which gives it one combination of linear and circular polarization or it can take the s path and emerge with the opposite combination of polarizations. When linear and elliptical polarizations combine they are called elliptical polarization. The two slightly divergent paths are taken as indicative of which slit the light went through as a particle but I think a more likely explanation is that they indicate which way the light went through the BBO crystal as a wave. The actual explanation is much more complicated because the light that enters the BBO crystal is light from a high powered UV laser and this same UV light emerges from the other side. A miniscule amount of the original light emerges as a spectrum of shorter wavelengths including a lot of IR. And a small amount of the emerging spectrum is in the red range. The light also passes through two more birefringent crystals and two color filters to remove all but the, by now, four beams of red light. It is the only the red light that is used for the experiment and for the later determination of which-path information. The which-path determination is made on the red light that went through the BBO crystal rather than on the UV light that went through the slits so I find the latter interpretation of which-path information questionable. I have mentioned the main experiment I have in mind several times as the Wheeler Delayed-Choice Quantum-Eraser experiment. There are a number of variations of this experiment from simple to complex but the one that is commonly referred as The Delayed-Choice Quantum Eraser is the Yoon-Ho Kim et al experiment (1999). I am familiar with Kim’s original article but lately the only references I have been able to find are behind a pay wall. Wikipedia does a good job of describing the experiment and has illustrations from the original article. There are also loads of videos on YouTube about the same experiment. I would also recommend the recent videos on YouTube by Don Lincoln of Fermi Labs for a nontechnical explanation of quantum eraser experiments given from the point of view of a particle physicist. (not my wave view) I also have a simple working model of the experiment that nearly anyone can try with commonly found materials. The interpretation is that if which-path information is somehow available, you don't get interference. If which-path is not available or has been destroyed you do get interference. If you do the test in duplicate with entangled particles where one test comes first without which-path information and another comes later with which-path information, you don't get interference in either.
-
I agree the relationship between an observation, mechanical or otherwise, and an experiment is not casual. The double-slit quantum-eraser experiments do involve polarizations and that is the type of experiments in question. People use polarization in the experiments as a means of "marking" the which-path information without disturbing the light paths by other means of observation. They are trying to show the causal effect between the availability of which-path information and the experimental results as described by Skully and Druhl. The theory is that an experiment potentially has multiple outcomes before it is observed. That is not my opinion.
-
We may be saying the same thing. If the experimental setup is such that it provides availability of which-way information, the results will show no interference. If the setup does not provide availability, there will be interference. The observer has nothing to do with the outcome. The conventional explanation holds that the observer can alter the results but that is not my view. The logic behind the conventional view is that, if the experiment is run in duplicate so the outcomes should be both with and without interference, the results are found to be identical for both experiments depending upon which results the observer chooses to examine first. That is the problem. “All this stuff” refers to a quote I posted from wiki. And needless assumptions is also my position. If I quote something from an outside source, that does not imply that I support it. I only do so to contrast my view with the usually more conventional. Did I not answer your questions or did you not read my answers? Tell me what I did not answer. I am sure you know light is slowed while passing through an optically dense material. If that material is a nonlinear crystal such as calcite, the electro plane will be slowed more upon entry than the magnetic plane giving the wave the properties of a spiral. This is circular polarization. If the light is a common source and it is passed through orthogonal polarizing filters, then it becomes a matter of polarization. The discussion is about why the two beams do not interfere.
-
That explanation works for me. I did answer your questions in the post directly following the one where you posted the pictures from the book citing Fresnel and Arago. In retrospect, I would change my answer about there being no such thing as planar light waves since the term “planar” includes light waves in parallel. So my answers are that there should be a Poisson dot in all three cases. Much was known about polarization in the 1800-1817 years beginning with the work of Enne Malus. Please read my answer this time- The implication is that an electric current can be used to slow the electrical plane of a transverse light wave as it travels through a transparent material to induce variable amounts of circular polarization. Or it can be used to rotate linearly polarized light. One gadget that can do this is called a Pockel’s cell. This has a number of practical uses such as modulating a light signal. That answer together with "swansot's" works for me. I have probably seen such diagrams but if you have a diagram showing how light passes through a nonlinear, birefringent crystal that would be helpful. It is my observation that none of the conclusions offered so far about why orthogonally polarized light beams do not interfere find application to explain the workings of the double slit experiment. The explanation favored by all experimenters is the one proposed by Scully and Druhl that the availability which-way information destroys interference. Here is a quote from wiki which is the typical of explanations found elsewhere. "The quantum eraser experiment is a variation of Thomas Young's classic double-slit experiment. It establishes that when action is taken to determine which of 2 slits a photon has passed through, the photon cannot interfere with itself. When a stream of photons is marked in this way, then the interference fringes characteristic of the Young experiment will not be seen. The experiment also creates situations in which a photon that has been "marked" to reveal through which slit it has passed can later be "unmarked." A photon that has been "marked" cannot interfere with itself and will not produce fringe patterns, but a photon that has been "marked" and then "unmarked" will interfere with itself and produce the fringes characteristic of Young's experiment.[1]" The Scully-Druhl hypothesis is supported by observations but I don’t find that it explains what is happening at the physical level and it suggests that the knowledge of the experimenter is instrumental in the outcome of an experiment. That should be hard to accept but it has come to be the popular explanation.
-
The question remains unchanged and it pertains to the Fresnel-Arago statement (1) from your book and I favor the wave theory as described by Fresnel and Arago as the most valid of the two possibilities that I listed but I am asking about your answer or that of anyone else who might have an opinion. Here is a repeat of the question. You posted a picture of two pages from a book. On page 358 “Polarized Light” it states: "(1) Two beams of light plane-polarized in mutually perpendicular planes do not produce interference fringes under any condition." Why do they not produce interference?
-
If you prefer, here is something more Socratic. You posted a picture of two pages from a book. On page 358 “Polarized Light” it states: "(1) Two beams of light plane-polarized in mutually perpendicular planes do not produce interference fringes under any condition." Why do they not produce interference? One possibility proposed by Fresnel and Arago is that the two beams interfere so rapidly and in such a random manner that the light is no longer coherent. Another possibility proposed by Scully and Druhl is that polarization places a which-path tag on the individual photons providing certainty of their positions and the mere possibility of obtaining such information destroys interference. Neither of these explanations is complete as stated. I am just suggesting possibilities but what would be your explanation? Also, the answer to the question is relevant to the Yoon Ho-Kim et al double slit experiment among others for which the Scully and Druhl explanation applies. https://en.wikipedia.org/wiki/Delayed-choice_quantum_eraser Quote from wiki- “ Significance" "This result is similar to that of the double-slit experiment, since interference is observed when it is not known from which slit the photon originates, while no interference is observed when the path is known.”