
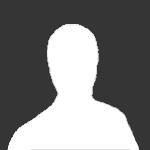
CPG
Members-
Posts
28 -
Joined
-
Last visited
Content Type
Profiles
Forums
Events
Everything posted by CPG
-
The basics are simple, but don't let that deceive you into believing you are an expert in the field. If there's one thing I've learned from being in a university department where my millisecond-level neuronal events are considered just as intensely as deep time, it's not to make claims stronger than I can support. We can talk theory, but the details inevitably get complicated.
-
How does the Facial Nerve actually work.
CPG replied to BMac's topic in Anatomy, Physiology and Neuroscience
Just thought I'd add that individual cells (including neurons and muscle cells) are sensitive to the frequency of their inputs. The way I think of this is to first consider the passive properties of cell membranes, namely its resistance and capacitance. Any given cell will have a resting permeability (or conductance), and the membrane behaves like a capacitor so the surface area of a cell membrane largely determines its capacitance. These two are in parallel with one another, so as in any basic RC circuit low frequencies exhibit more influence on the membrane potential, and higher frequencies have less influence. With this knowledge you can generate a curve that describes impedance as a function of the frequency of current input (e.g. synaptic currents). Now let's add in the active properties of excitable cells: ion channels that are gated dynamically by factors like membrane voltage and intracellular calcium concentration. Each of these ion channel types will have its own specific voltages at which it opens and closes (activation, deactivation, inactivation, deinactivation). This opening and closing is due to conformational changes in the channel proteins, and these conformational changes have their own time constants. When all this is said and done, that curve you'd expect based on the passive properties will be re-shaped by a cell's active properties and the cell of interest will display a unique impedance profile for how effective particular input frequencies affect the membrane voltage. If that sounds really complex, you're right. But it's also what give these types of cells an incredible diversity in their ability to integrate signals over time and ultimately determine cell-type specific activity in response to a given signal. -
That's disappointing. I had hoped the responses to the OP would start a nice scientific discussion. The feedback from the initial question seems to have been completely ignored. I'm fairly open to wherever discussions progress. Non-sequiturs aside, some sort of reasoning should be provided to support the claims in sentences 1,2, and 4.
-
Pain can be a tricky thing to talk about, and a more focused question could facilitate a better discussion. A few considerations to move things along in a productive direction: 1) When you say the vast majority experience little to tolerable levels of pain, it sounds as if you have a specific type of injury in mind or at least would be able to provide an example. The type of injury, and to what part of the body, could be very useful as a starting place because then we could discuss the relevant neuroanatomical structures and functional networks involved in concrete terms. We should also be very deliberate with our language in discussing nociception (sensory signals encoding information about harmful stimuli coming from the periphery), as opposed to the conscious perception of pain and the unpleasant subjective experience that occurs when these signals are received and processed in the brain. 2) Are we talking about acute pain at the moment of injury and the immediate aftermath, or longer term pain such as chronic and persistent pain during healing and recovery (or even beyond)? The considerations associated with these two are quite different. 3) "Why" questions are notoriously difficult to answer. I have assumed you're looking for an explanation along the lines of a physiological contrast between a person who experiences a lot of pain and one who doesn't. But at the same time your question could also be interpreted as more ontological; What adaptive value and evolutionary consequences are associated with various types of nociception and/or pain - and could that explain the population-level variability that we observe? 4) I'm also curious whether you're describing a spectrum of pain that serves a purpose, or if your "extreme pain" case is really more of a pathology. When I say "purpose" I'm referring to the instructive and even punishing aspect of pain that can be useful if it alters your behavior to avoid repeating or exacerbating tissue damage.
-
Human tactile sensing data, anyone can help?
CPG replied to al777's topic in Anatomy, Physiology and Neuroscience
There has been lots of research in this area. Could you elaborate about the scope of your interest or be more specific about values you're looking for? Are you exclusively focused on mechanosensation in the human hand? I would suggest looking into time-varying stimuli like changes in pressure and vibration. These are important in perceiving things like texture and are ultimately based in the type of parameter you seem to be looking for. One suggestion I can think of right now is that you take a look at "Detection of Vibration Transmitted through an Object Grasped in the Hand" by Brisben et al. 1999. I would guess that could add to your parameter set. One reason you may be having trouble is that the value you're looking for may not be a single fixed value, but vary with other factors like behavior that can modulate sensory discrimination. It depends how in-depth you want to go with your essay, but I applaud the effort. There are reasons it's been a huge challenge in robotics to build a robotic hand that can recapitulate the sensorimotor dexterity of a human hand! Probably the best suggestion I can offer is to find a comprehensive review article on Pubmed, and follow the citation trail to the original experimental work as necessary. -
Not to derail the hard work you've already put in, but for your consideration if this continues to be an issue... If you're worried about false positives and troubleshooting isn't going well, do you have the option available to you to take a different approach to the same question? I don't know what would suit your purposes well, but assuming you're just looking for protein-protein interactions there is FRET, co-IP, BiFC / DERB, that sort of thing?
-
I'll offer up a disclaimer that this is not my area of expertise, but it's a good question worth addressing so I'll give it a shot The common thread here is that both epigenetic mechanisms affect what portions of the genome are accessible for transcription, but they tell you different things. I think there are a variety of reasons for the discrepancy you noted on Pubmed, but as you suggested the available assays and what they they tell you certainly play a role. The history of when epigenetic markers were discovered, and how useful they are would also factor in. Sometimes what is "trending" and gaining interest can factor in. (Suggestion: Try your Pubmed search again and look at the histogram that appears to the right which shows publications by year that match your query.) Eventually some assays gain enough momentum to become widespread lab techniques. This particular field that you are inquiring about is in a state where there are some well-established assays and others that are constantly changing with fancy new technology. DNA methylation assays would be in the former category. Assays of methylation can tell you precise information about exactly which base pairs are methylated in a given sample. Our capabilities with genomic sequencing are becoming more advanced and cheaper all the time. The situation is much like Moore's Law for biotechnology in that respect. One common technique to do this uses the fact that methylation often occurs at Cytosine residues that are next to Guanine, so-called "CpG" sites. If you're familiar with PCR, it requires a little extra step where you treat the DNA sample with sodium bisulfite. If the Cytosine is not methylated, it will be converted to Uracil. If it is methylated (5-methylCytosine), it is not going to be converted. Then you're able to amplify your bisulfite-treated DNA using PCR and sequence it. Once you have that sequence, you can align your bisulfite-treated sample with a reference genome or untreated sample and you can see exactly which sites were converted and which were not converted. By inference, you now have very specific information about the epigenetic markers' position and can begin to interpret more about how that impacts gene expression at that locus. In a way, it gives you a nice clear "snapshot" of the state of DNA. I have never performed this technique myself, but my impression is that it is easier and cheaper than a lot of histone modification assays. When dealing with histone modifications (such as acetylation), you are dealing with chromatin and have to deal with more macromolecular complexes that are important and ultimately can affect the accessibility of large portions of the genome for transcription. The 3D structure of this lends itself to a more difficult interpretation than the linear base pair approach as in classical genetics. Notice that you can look at DNA methylation from this perspective. I think as a result, there tend to be other assays people often run first that don't zero in on histone acetylation per se. A common approach to identifying regions of the genome that are made either more or less accessible by the state of the chromatin would be something like chromatin immunoprecipitation (ChIP) which may not necessarily tell you specifically about acetylation. There can be many other chemical modifications involved (palmitoylation, methylation, sumoylation...) that may not be resolved by this method. Essentially, here is the basis for this assay: because histones are proteins that interact with DNA they can be chemically cross-linked so that they stay bound together. You would then be able to use enzymes to digest the exposed portions. Then using antibodies against histones, you precipitate out the regions that are undigested and pull down the undigested DNA along with the antibodies. At this point, you can determine which portions of the genome have been made inaccessible by chromatin modifications and which were exposed to enzymatic degradation. If you're interested specifically in histone acetylation you would then proceed accordingly from there, but off the top of my head I'm afraid I'm not able to be a competent guide through this portion. Another broad route to go would be to look for expression of the enzymes responsible (Histone acetyltransferases / histone deacetylases). This alone could indicate their presence or absence but would not be very helpful in telling you where throughout the genome they are acting. The technology for this stuff is rapidly advancing so I am pretty sure my knowledge is lagging behind the times. I may ask a friend and colleague who does this stuff for a living to offer her two cents if she has the time.
-
How do many sounds get received by an ear?
CPG replied to Graeme M's topic in Anatomy, Physiology and Neuroscience
True, I did not intend to give the impression that the fluid of the inner ear is continuous with the outside. For anyone wishing to visualize why this anatomical clarification matters, here's a brief animation of the physiology at work: http://blausen.com/?Topic=8814 -
How do many sounds get received by an ear?
CPG replied to Graeme M's topic in Anatomy, Physiology and Neuroscience
I'll try to add some anatomical perspective that I think could help tie things together with some of the physical and mathematical perspective on acoustics that has already been provided (good posts!). The mechanical vibrations of the hair cells along the cochlea are able to open ion channels in order to transduce vibration into a neural (electrical) signal. http://www.nature.com/nrn/journal/v15/n9/images/nrn3786-f3.jpg Hudspeth, A. J. Integrating the active process of hair cells with cochlear function. Nat. Rev. Neurosci. 15, 600–614 (2014). Let's zoom out from the cellular level to see the structure and layout of the cochlea. Different locations along the structure are differentially sensitive to the frequency content of these vibrations. This is mostly a result of the cochlear structure rather than having hair cells that are sensitive to different frequencies. I'm oversimplifying a bit here but you can approximate it like so: Image Source: https://sites.google.com/site/jayanthinyswebsite/_/rsrc/1380059170661/workshops/different%20aspects%20of%20organ%20of%20Corti.png These neurons then project sensory information to the brain. If you're already familiar with how our sense of touch is somatotopically organized in the brain (different regions of the brain represent different parts of the body), this acoustic information is handled in a similar way. The auditory cortex in the brain has a tonotopic organization that allows the incoming signals to be processed in different places, and you end up with an organization in the brain something like this: Image source, University of Calgary: http://ucalgary.ca/pip369/mod6/sound/auditorybrain A few side notes: - Sound does not travel in air as it does the aqueous environment of the cochlea. The bones of the middle ear are needed to perform impedance matching, so that vibrations resonating at the eardrum will be mechanically transduced to vibrate appropriately in fluid of the inner ear. - As long as you're thinking about the structure of the cochlea, notice that the high-frequency hearing range is towards the opening where all sounds must pass through. As people age, we tend to lose our hearing most dramatically from this end, but it is very loud and low frequency vibrations that do the most damage. (ie blasting the bass too loudly in your headphones when you listen to music). - I tried to provide a simplified overview, but I would like to take this opportunity to apologize to nature for glossing over her staggering complexity and elegant solutions to so many real-world problems in acoustic perception. CPG Edit to add: The first image I linked is visible to anyone visiting the nature website, but unfortunately the full text of that review is not. Sorry -
If anyone does decide to try the proposed experiment, make sure you are starting with seeds that are actually viable. Many of us have become accustomed to only eating watermelons with seeds that are sterile to begin with. These would be the seedless watermelons, which mainly produce those white soft seeds along with the occasional dark hard seed. These plants are made by crossing diploid and tetraploid plants. That results in triploid offspring, so naturally these plants with 3 copies of each chromosome have trouble evenly dividing them into two cells as they undergo meiosis to form gametes. I have heard that the diploid lines are the heartiest and easiest to maintain... Happy experimenting!!
-
Extracellular potassium increase
CPG replied to Function's topic in Anatomy, Physiology and Neuroscience
The convention is to define the extracellular solution as 0 Volts and compare the intracellular solution to that. So you are correct if you were thinking that adding solely positive charges (eg pure potassium) and nothing else to the extracellular solution it would change the net charge and cause the membrane potential to be hyperpolarized. Trying to do that would be dangerous, so please don't try it on me! See this youtube video for what that would look like. I think by focusing the question on one ion, it is easy to temporarily forget that you're adding a potassium salt that has an equal number of anions (Cl-) for every cation (K+). The transmembrane voltage at any given instant is a result of differential distribution of charged particles on different sides of the membrane. The net charge inside versus the net charge outside is what is going to determine the charges that get distributed across the membrane. In the solution you have added the (+) and (-) charges are in equal proportion so the net charge (Q) of the extracellular solution as a whole does not change. If you had an intracellular electrode recording from inside a cell, you would see a slow and steady depolarization of the membrane potential. The reason for this would be that under normal conditions the membrane would be most permeable to K+, so it would exert the most influence on membrane potential. With the concentration change, you have shifted the point at which it reaches equilibrium. How long this would take would mainly depend on blood circulation and diffusion. Honestly, I think the best way to get a feel for this (if you don't have an electrophysiology rig and animals at your disposal) is to check out this simulator. Launch it, and to get conditions similar to the human body choose Goldman @ 37degC. Once you have that open, you can fiddle with concentrations and permeability and see what happens to the membrane potential of a simulated cell. It's very cool and once you figure out what the buttons do it makes some complex ideas very intuitive. Check it out! http://www.nernstgoldman.physiology.arizona.edu/ -
Extracellular potassium increase
CPG replied to Function's topic in Anatomy, Physiology and Neuroscience
Your question is confusing and not very specific, making it difficult to provide a direct answer. What are we injecting K+ into? Intracellularly or to the extracellular environment (blood, artificial physiological saline, etc)? If it's the extracellular environment, what is the concentration of the K+ solution you are adding? What is the volume of the solution to which you are adding it... etc. Perhaps I'm not understanding correctly but I fail to see why cells would hyperpolarize at the moment of injection. Help me out here, and I can probably clear up some confusion. Regardless of the specifics, this should help with a general understanding that should help: Start by examining the Nernst Potential. This allows you to predict the equilibrium potential (membrane potential at equilibrium, also called Reversal Potential) for a single type of ion: in this case, K+. This equation is based on the relevant biophysics and as long as we assume temperature does not change, all the values are constants as listed in the wiki link. The variables here are extracellular ion concentration and intracellular ion concentration. When K+ channels are open, they will move the membrane potential towards its equilibrium potential. You can see in the equation that increasing [K+] outside the cell will shift the equilibrium potential to a more positive voltage. Note: You mentioned Goldman, so if you're dealing with the Goldman-Hodgkin-Katz equation, you can think of that as essentially this same concept for multiple ions. At a hyperpolarized resting potential, neurons tend to be most permeable to K+, so the reversal potential of K+ has the most influence over the resting potential. With the shift in the potassium equilibrium potential (EK) K+ flux across the membrane will move the potential to this new value, which is a more depolarized potential. -
Fourier transformations are strange to look at at first, I'll admit. If you want to calculate the frequency of an event occuring, the straightforward measurement would be to count how many times the event occurs and divide it by the total amount of time, right? However, in this situation, what they want to do is look at different frequency inputs a neuron might receive (X axis), and how effective that frequency is at changing the membrane potential (Y - axis). The goal to describe the neuron's response to different frequencies of input. With frequency as our independent variable and impedance as the dependent variable you can now see that time is removed from consideration - and yet we are still dealing with frequency... what the heck? The Fourier transform is a way to take any signal (let's say it's a recording of current injected into a cell) and break it down by how much of that signal is made up of a given frequency. Let's say hypothetically you had a perfect sine wave with a nice steady 1Hz frequency. This means it goes through one full cycle - peak to peak - exactly once per second. The most abundant frequency present in a signal will turn out to be the peak on a Fourier transform so your ideal sine wave would show a peak at 1Hz and nowhere else. In the process of doing that, time actually gets dropped out so it's a mathematical way to look at frequency without consideration of the time domain. But where does it come from? There are many different ways to actually do the math but I just want to stimulate some intuition for it here. If you want to take a signal and calculate frequency, sticking with our sine wave, we can take the 2nd derivative at any given point. The rate of change of the slope gives you an instantaneous frequency. Do this for the whole signal (ie the whole current injection) and tally up how much you get for each frequency. That is the "power" for each frequency. If you are injecting current you will get a voltage response from the cell and do the same transformation. This can give you a profile of frequency-specific impedance. Here's how and why that matters: Tying it together with my previous post: a) Ohm's law tells us that Voltage/Current = Resistance b) Take the Fourier Transform of Voltage, divide that by the Fourier Transform of the current the cell receives, and that gives you a curve that describes the frequency-specific resistance (Impedance) of the neuronal membrane. If there is a peak in impedance at a specific frequency, biological input at that frequency would be more effective at changing membrane potential than at other frequencies and thus more effectively drive that cell. Tying it together with the rest of the thread: a) I originally posted that link because it seemed to someone that neuronal output can't carry a "signature" or the richness of information that they do. b) Neurons are capable of firing at different frequencies, so it's not unfair to call a particular output frequency a "signature" that helps describe a specific stimulus. I have heard pain researchers say that other types of sensory fibers (ie carrying information from touch or temperature or something else) can also be interpreted as a painful stimulus by the brain if it comes at a particular frequency - even though it's coming from nerves that usually provides a very different sort of feedback. I would have to do some searching to find citations in the literature documenting this exact phenomenon with respect to pain, but I have been told that this occurs. True, that way of answering leaves some evidence to be desired, but having said all this I'm curious and intend to look around the literature when I get the chance and see what I can find out. I don't want to forget about posting, so if anyone is curious or skeptical, let me know and I'll be happy to reply after I read a few papers. Just remind me so I don't forget!
-
Action potentials can carry far more information than the binary yes/no implied above. Even if we are only considering all-or-none events at a single synapse, you'd also always be dealing with the precise timing and rate at which they occur. Firing at different frequencies (2 Hz, 50 Hz, etc) can have entirely different effects on the post-synaptic neuron, and can make the difference in how the signal is processed (ie filtered out or amplified). The synaptic as well as intrinsic properties of the neurons which are sending or receiving a signal can display complex temporal dynamics that are fundamental to neuronal signal processing. The paper linked below makes for a nice theoretical introduction with its graphical depictions which in my opinion really go a long way towards assisting an understanding of how neurons can have such "frequency preference" characteristics. Bursts as a unit of neural information: selective communication via resonance (Izhikevich et al. 2003) http://www.ncbi.nlm.nih.gov/pubmed/12591219
-
That's a really complex topic and an interesting question. The conceptual part that seems mot difficult to me is the brain "mapping" the entire body and the space around it. If you first look at how such body mapping is thought to occur, localization intuitively seems like a straightforward process. There is a book by Sandra Blakeslee and Matthew Blakeslee that deals with this topic really well. Even shorter version than the book, Ginger Campbell's "Brain Science Podcast" has an episode or two about that book and interviewing Sandra Blakeslee. Those podcasts tend to be an hour long or so. Depends how deeply you want to get into the topic, but those are my recommendations Also, check out the wiki article on "referred pain". Similar to the phantom limb, I think examples of this process gone wrong that are informative about how it works. http://en.wikipedia.org/wiki/Referred_pain
-
Do you mean you want to train the BCI based on the subject thinking about a particular motor pattern? It sounds like your question is essentially about the detection and decoding of neuronal signals. To calculate the physical limits of detection you need a lot of information about the sensitivity, placement, sampling frequency, etc. of your electrodes. Then we need to know what algorithms are being employed to decode the signals in a meaningful way. (I'm guessing some of these specifications are available at some estimation from the manufacturer, while others may not be. For example, they might not specify regarding proprietary features of their technology). Some motor outputs may have very broad and easily detectable signals, but others might be harder to detect and below the threshold of your detection, or far too variable (trial-to-trial // person-to-person) to be consistently trained and recognized by the BCI. I don't think we on this forum have enough information to tell you what you should be able to detect with your current machinery, but you can experimentally employ your system to find out the limits of these parameters for yourself. Then you would need to determine whether what you have is sufficient or if you need to improve your resolution, processing, etc. Also, if you want the BCI to have more than one of these, you'd also want to be aware of potential overlaps in the computational "decoding", and make sure that the patterns you want to recognize are not only recognizable, but also distinguishable from all others.
-
I have to ask an important question about the question in the OP. A typical sodium channel that contributes to action potential conductances has more than 1 "closed state". It can be closed because the channel is inactivated or because it has deactivated (this technicality may sound silly at first but they are mechanically and functionally very different) - so which is the drug preventing? Did the question specify? I'm going to reply as if the channels cannot inactivate because that's what I would guess is meant here. Normally in an action potential it is inactivation that is responsible for closing Na+ channels. If the drug is preventing inactivation (there are definitely drugs that do this, and some very effective ones like pyrethroids have been commonly used as insecticides because they do this to insects. Once their nerve cells are excited they can't repolarize and so you've effectively shut down the nervous system). If you don't mind a few simple algebraic equations, it may help out a lot here. A brief description and then the math. More sodium channels being open makes the membrane more conductive to sodium and thus would drive the membrane potential towards ENa (aka the equilibrium potential for sodium). The driving force for an ion, Na+ in this case, is the difference between the membrane potential at any given moment the ion's equilibrium potential (Vm - Veq). Conductance (G) is another way of describing the same property as resistance ® - it's just that with conductance we're concerned with how easy it is to pass current (I) instead of how strong the impediment to current is. Here comes the reason for all that setup. The current flowing across the membrane is equal to the conductance times the driving force. I=G*(Vm-Veq) So let's say your drug prevents inactivation of sodium channels, depolarization causes a positive feedback leading to further depolarization. However, since the Na+ channels stay open GNa remains high and continues to drive the membrane potential towards ENa which would typically be a very positive voltage. Your K+ channels also open in response to depolarization, albeit more slowly. As they open, K+ currents drive the membrane potential towards EK (hyperpolarizing). At this point because the sodium channels have been doing their thing, you're probably very far away from EK , so the driving force for K+ would start off very strong. These two currents are now opposing one another, and as K+ current drives the membrane potential down, you get farther away from ENa, and thus Na+ currents increase in magnitude. Likewise, as you approach EK, K+ currents decrease in magnitude (assuming no conductance changes). Picture two of the equation above, but one for each ion: INa = GNa (Vm - ENa) IK = GK (Vm - EK) Hopefully with this illustration you can see that as long as both channel types remain open, the membrane potential would equilibrate somewere between these two values, at a membrane voltage where the currents are equal and opposite. This point is likely to be above the voltage where activation / deactivation takes place. If that lost you at any point it's good to always keep Ohm's Law in mind. That will remind you what's going to happen to the membrane potential whenever current is flowing. V=IR (or V=I/G) Depending on the level of complexity of your studies, there may be more involved, but my best guess is that this is generally the logic your instructor had in mind. The electrophysiologist in me wants to add a list of caveats ("ifs ands & buts"), it's probably best to stop here and ask if what I've laid out makes sense so far? (The last part of your question does make me wonder in what depth your instructor went, because the activation of channels can be described by probablistic functions and we might start worrying about conductance changes due to the voltage. The typical approximations of this have different functions for activation of Na+ channels and K+ channels. However, as long as the voltage remains well above the activation threshold, you can probably assume that the fraction of channels that are closed is negligible.)
-
Hi everyone! New to the forum. YFG question!
CPG replied to jasrod829's topic in Biochemistry and Molecular Biology
You may find it a useful starting place to glance at a quick resource for what has been done experimentally, peer-review, and published. Since this is Drosophila, you can head on over to the appropriate page on FlyBase. There's some characterization there that will give you some back ground info, including expression profiles. In my opinion anything there can only become "Your Favorite Gene" when you've stumbled across a reason to care about it and think its cool. (I'm assuming that's what you meant by "YFG"). By its nature, that is subjective, so I'd suggest scanning some of the expression info in the link until something pops out at you. I find molecular biology experiments interesting when they stem from a biological question. For example, if you find out what a protein DOES you might end up suspecting that it could be useful for the cell to produce under certain conditions, but undesirable in other conditions. Is this the biological strategy that is used? Is expression turned on and off the way you would predict? If you can provide the conditions and compare groups you can ask that question (aka do the experiment). As another example, you might ask whether a protein known to be in the cell is localized to a specific place within the cell in order to do its job. If you suspect that the protein interacts directly with DNA, then you tag it with GFP and the nucleus - but nothing outside the nucleus - lights up green that subcellular localization would be consistent with your hypothesis about where it functions. I suspect if you're using GFP you'll have access to some sort of fluorescence microscopy in order to collect data from the experiments, in which case you'd want to make sure you have the necessary resolution to see that kind of thing before you design an experiment. -
Very interesting and puzzling situation. This is all armchair speculation, but if I were to try to figure out what might be responsible for phenomena as you've described them, one area I'd be inclined to learn more about and address with an appropriate medical professional would be common opponent processes in the visual system. This would be accomplished by the neural circuitry throughout the visual system - all the way from the retina and extending throughout the visual cortex. For example, a blue vs yellow center-surround antagonism is a generally accepted feature of the magnocellular pathway the visual system. A similar red-green antagonism is well described in the parvocellular pathway and you may find more abundant resources detailing how that works. There would of course be many other features of this condition to look into as well, including the signal amplification and dampening that occurs to aid in the discrimination of boundaries / contours in the visual landscape, etc. Understanding the neural circuitry of the visual system happens to be an area where a lot of academic progress is being made, which means there is a lot of information out there. That can be both good and bad when you try to sift through it all, though. I just put a few things in bold/italics that might make for good search terms if you want to seek out more comprehensive resources. Wanted to add: If finding good resources explaining the anatomy & physiology I mentioned proves to be tricky, let me know and I'll try to find something good in the public domain next time I drop by here
-
Heavy metal, receptor blockage?
CPG replied to DevilSolution's topic in Anatomy, Physiology and Neuroscience
Calcium would normally be able to flow across the cell membrane through protein complexes that form calcium channels. Physically occluding the pores essentially means Cd2+ gets stuck in these structures and thus prevents calcium from flowing through the channels. If calcium cannot flow, then any voltage changes that would result from calcium currents will not happen, and many other intracellular processes dependent upon calcium influx will not happen. The list of things intracellular Ca2+ does would be a very long one. By "taking calcium currents out of the equation" I just mean that it can help achieve cleaner results in experimental electrophysiology. There are actual equations for this stuff, but that's typically when the eyes begin to glaze over so instead, to illustrate... Let's say I am interested in a particular potassium current, and I want to characterize the role of electrical current flowing across a cell membrane that is due to voltage-gated potassium channels. It would be very helpful, then, to block any other possible sources of current aside from the potassium current I care about. In order to do that I might add cadmium to the solution around the cell to block Ca2+ channels, and then I add other substances to block sodium channels and any other path for charged particles to move across the membrane except those potassium channels that I care about. Then if I measure current flow across that cell membrane, I can be confident it is the one I wanted to characterize and the properties of that current are not contaminated by calcium currents or other currents. http://en.wikipedia.org/wiki/File:1K4C.png A visual aid from wikipedia to help in thinking about ions moving across a channel, and how the molecular properties of ion channels provide their selectivity. This is a potassium channel illustrated here (chosen because their structure & function is particularly well understood), so you'd find a slightly different structure providing the selectivity in a Ca2+ channel. These "selectivity filters" in ion channels tend to be highly conserved and are targets for many toxins, poisons, etc. In this case, I was referring to Cd2+ being similar enough to Ca2+ to get into the pore / selectivity filter, but different enough that it can't pass through and instead it remains in the pore and prevents Ca2+ from passing through. -
Heavy metal, receptor blockage?
CPG replied to DevilSolution's topic in Anatomy, Physiology and Neuroscience
As an aside from the OP's reference to synaptic communication, certain heavy metal ions (I'm thinking particularly of divalent cations here) may alter the intrinsic excitability of individual neurons of interest due to their molecular interactions with voltage-gated ion channels. For example, I regularly use cadmium because in the neural networks I study, it physically occludes the pores of the calcium channels present and effectively takes Calcium currents out of the equation. It's useful to do this kind of manipulation in experimental electrophysiology because you can then measure other membrane currents without the contamination of calcium flux and its effects. I just wanted to add the important bit about effects on non-synaptic neuronal intrinsic properties because it seemed that only chemical synaptic ionotropic receptors were really being considered thus far, which would ignore how individual neurons process whatever inputs they do receive. I'm afraid I can't speak very knowledgeably to human health consequences, concentration-dependent effects, half-life in vivo, or other concerns in the OP. However, I do agree with what is said above about the complexity you're likely to deal with at that level and the general promiscuity of biomolecular interactions. Just adding another potential neuronal effect to the list. -
Nerve conduction study questions...
CPG replied to jerrystraub's topic in Anatomy, Physiology and Neuroscience
-
Nerve conduction study questions...
CPG replied to jerrystraub's topic in Anatomy, Physiology and Neuroscience
Thank you for the heads up, Ringer. Those images are not mine, I guess I was thinking the embedded hyperlink would show the source but I will make it clear later when I get back to my computer. Posting from my phone now so it would be tricky to cite. Thanks Edit: I could not edit the original post, I assume because too much time has passed since it was posted, but it is reproduced below giving credit for the images where it is due. Any help from a moderator in fixing the problem would be much appreciated. I seem to have made a mess of this one! -
Neuroscience Teaching tools/learning materials
CPG replied to AJS's topic in Anatomy, Physiology and Neuroscience
The Society for Neuroscience, along with The Kavli Foundation and the Gatsby Charitable Foundation, maintain a site that has information accessible to the layperson and is probably a good place to start for an answer to general questions about the brain. An excellent go-to source if you are generally curious about anything brain-related. One thing I do like in particular is the way it address certain "brain myths" that people hear and wonder if it is true (there is a hyperlink at the top of the page that says "neuromyths" as of the date I made this post): http://www.brainfacts.org/ -
Nerve conduction study questions...
CPG replied to jerrystraub's topic in Anatomy, Physiology and Neuroscience
The membrane itself acts has a very high resistance (and also capacitance but let's disregard that for now). By Ohm's law, if you pass current across a resistor, you develop a voltage across that resistor. In this case, particularly across cell membranes rather than a more diffuse voltage gradient. In stimulating extracellularly, there are a number of variations that can be done (anodal versus cathodal stimulation for example), which would have a different effect on WHERE a given amount of current is able to elicit an action potential. The X-axis below shows 0 as the site of stimulation and the Y-axis shows the pattern of voltage change that would be elicited from such a stimulating pulse. The graph below that helps to illustrate how the variables of current polarity and distance would affect the region in which stimulation to threshold would be achieved. You may also note from the first image above that other considerations can come into play for specificity of stimulation (for example arranging stimulation so that if an axon is depolarized at one location, it is hyperpolarized at another so any action potential that is started would simply be unable to propagate. Alongside that, the strength of the stimulus can be varied, and all else being equal it is generally easier to recruit large-diameter fibers than smaller-diameter fibers. It sounds like your device stimulates small fibers. Are they the only ones there, or are there also larger fibers present? My understanding is that there are ways to deal with it if there are larger fibers - for example taking advantage of the fact that their conduction velocity would be faster, and then strategically hyperpolarizing the nerve so that it interferes with their more quickly propagating action potentials, but the hyperpolarization which aborts their action potentials is gone by the time the slower-conducting action potentials of smaller-diameter fibers reach that region. I don't know specifically about the device you're describing but these general principles are presumable at play somehow. Hope that helps a bit. My apologies if the diagrams I chose are too much on the engineering side...